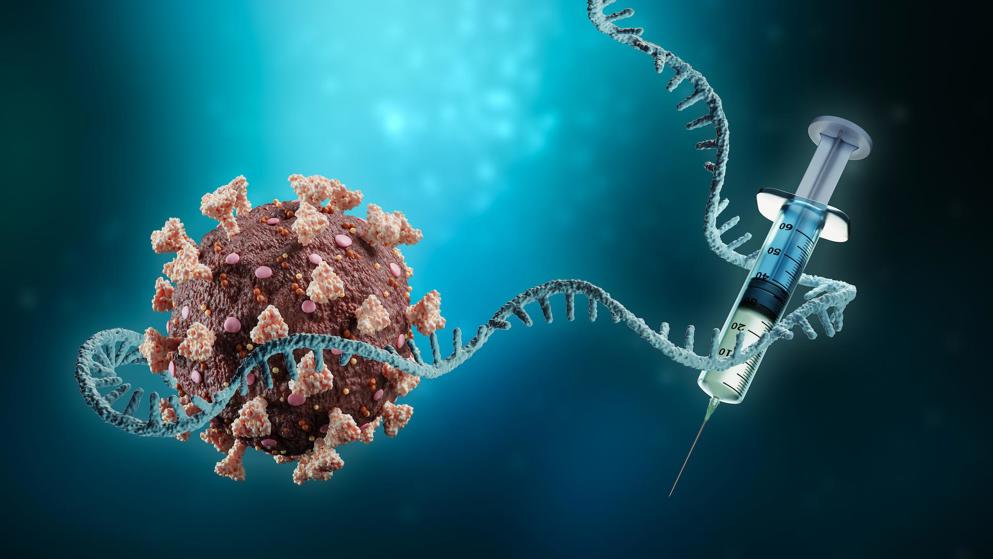
The future of vaccination in a post-COVID-19 world
In 2019, the WHO estimated that vaccines were responsible for saving 4 to 5 million lives a year globally1. In 2020, we witnessed a coronavirus disrupt the planet in a way no pathogen has before2. The full-scale assault that followed led to the most rapid development of a vaccine to date3,4. The COVID-19 pandemic has instilled optimism in many that the development of novel and improved vaccines against other pathogens responsible for major public health issues is more achievable now than ever4,5.
With continued research efforts, soon it may be possible to:
- Develop novel vaccines against pathogens for which there is currently no available vaccine (e.g., chronic infections like human immunodeficiency virus [HIV] and hepatitis C)6,7
- Improve the efficacy and/or uptake of existing vaccines for diseases that are not well-managed (e.g., human papillomavirus [HPV])8
- Enhance the efficacy and/or uptake of existing vaccines for well-managed diseases and potentially eradicate these infections completely (e.g., acute infections like measles, mumps, rubella, varicella, herpes zoster)9–11
Here we review recent advances, key areas for improvement, and future directions in the technology and clinical application of vaccines.
mRNA vaccines
The first vaccine to be approved against COVID-19 was also the first prophylactic mRNA vaccine to be developed5. The unprecedented success has largely been attributed to the recent advances in mRNA delivery systems12.
mRNA vaccines have been demonstrated to be highly effective, meet the necessary safety criteria, and have numerous advantages as a vaccine strategy13. As the mechanism of action is contained in the cytosol of the cells, mRNA vaccines do not have the concerns of genomic disruption and off-target effects as with DNA vaccines4. Modification at the nucleic acid level is easy and can improve the half-life and safety of the vaccine4. Production of mRNA vaccines is rapid, straightforward, and easily scalable4. Hence, the potential for developing mRNA vaccines against other diseases is strong and investigations are underway to develop vaccines against many acute and chronic infections including influenza, HIV, zika virus and dengue fever4.
We are likely to see more mRNA vaccines in the future, however, the technology is not without flaws and can be improved4,5. Increasing the targeting of dendritic cells via surface conjugation of specific ligands could improve vaccine efficacy4. Alternative mRNA delivery materials could improve vaccine safety12. In addition, current mRNA vaccines require storage in low temperatures making transport and administration of doses challenging, particularly for developing countries with warmer climates4.
Some investigations have shown success in animal models using an mRNA vaccine without any delivery system4,12. These ‘self-amplifying’ mRNA vaccines contain mRNA encoding an alphavirus RNA-dependent RNA polymerase, plus a second mRNA that encodes the antigen of interest12. The alphavirus component enables its own replication in the cytoplasm12. Self-amplifying (or self-replicating) mRNA vaccines may represent the next new generation of mRNA vaccines as they allow a sustained immune response, have a simplified production method, and it could mean the vaccine does not have to be stored in low temperatures4,12.
Use of CRISPR/Cas9
Currently, the use of the CRISPR/Cas9-based gene editing technology with viruses sits within the basic research space14. In comparison with other gene editing techniques, CRISPR/Cas9 is more efficient, specific, versatile, flexible, simple, and cost-effective14. As such, there is little doubt that this technology provides endless possibilities14. One of those possibilities is to modify the genome of a pathogen by gene deletion, substitution, and/or insertion to create vaccine candidates14. The editing of functional genes can also facilitate our understanding of viral pathogenesis and viral-host interactions14.
Several anti-viral vaccines for animals have been developed using the CRISPR/Cas9 system14. However, a long-term observation period is required to assess the editing efficiency and stability required, so whilst its feasible, it may be some time before we see a potential clinical application in humans14.
Vaccine awareness and acceptance
For vaccines to have an impact in the real world, it is essential for the public to be aware of what vaccines are available, and the associated benefits and risks15. As multiple studies have shown, poor education and lack of knowledge are frequently to blame for individuals not vaccinating16. In addition, we also frequently see the spread of misinformation about vaccines15.
Unfortunately, the relationship between knowledge and vaccine acceptance is not one of direct correlation so simply providing more information to the public will not automatically improve acceptance16. This is a key area for improvement in the vaccine field. What we may see more of in the future is the utilisation of risk communication strategies15. Healthcare professionals are in an excellent position to utilise these strategies, discuss the benefits and risks of vaccines with patients, and address any misconceptions15. Managing risk communication is particularly important because adverse events associated with vaccination represent a major cause of vaccine hesitancy15.
Vaccine hesitancy is a growing concern and was identified by the WHO as one of the top ten threats to global public health even before the COVID-19 pandemic17. Individuals who are vaccine-hesitant may delay or be reluctant to accept some or all vaccines15. Such hesitancy often reflects legitimate doubts about vaccine safety and/or more general concerns about the pharmaceutical industry, governments, and healthcare professionals15. In the future, we may see stronger policies for mandatory immunisation policies15. Whilst mandatory vaccines have been shown to be effective in increasing vaccine uptake, vaccine hesitancy is not directly addressed and resides15.
Vaccine adherence
The typical intramuscular route of administration for almost all vaccines causes localised pain and often multiple doses are required for full protection which can affect patient adherence to an immunisation schedule18. In addition, individuals who are needle-phobic may be vaccine-hesitant19.
The nasal influenza vaccine and oral polio vaccine are among the few non-intramuscular vaccines that exist and have been incredibly successful in increasing vaccine adherence18. Many clinical trials with vaccines using pulmonary or nasal delivery systems are underway, with these trials targeting acute and chronic infections including meningitis, influenza, respiratory syncytial virus, and tuberculosis18. Oral vaccines are probably the most patient-friendly and research is underway to bypass the challenges of developing oral vaccines against other infectious diseases18,20. Topical or intradermal routes, including the use of microneedles, are also under investigation18. Hence, in the future, it is likely that more vaccines that use a non-intramuscular route of administration will be availiable18.
Targets for 2030
The World Health Organization’s Immunisation Agenda 2030 sets the strategy to achieve the vision of ‘a world where everyone, everywhere, at every age fully benefits from vaccines for good health and well-being’21. Disease-specific targets have also been set, for example, to eliminate hepatitis C globally by 203022. Learnings from COVID-19 research can help us to achieve these targets and have turbocharged the development of vaccines against other infectious diseases4,5. Whilst the future of vaccination is promising, it depends on the success of continued medical research and increased vaccine acceptance.
References
- World Health Organization. Immunization. 2019. https://www.who.int/news-room/facts-in-pictures/detail/immunization. Accessed 19 April 2023.
- Woc-Colburn L, Godinez D. Lockdown as a public health measure. In: COVID-19 Pandemic: Lessons from the Frontline. 2021. Elsevier: 133–136.
- Chaudhary N, Weissman D, Whitehead KA. mRNA vaccines for infectious diseases: principles, delivery and clinical translation. Nature Reviews Drug Discovery. 2021;20(11):817–838.
- Jain S, Venkataraman A, Wechsler ME, Peppas NA. Messenger RNA-based vaccines: Past, present, and future directions in the context of the COVID-19 pandemic. Advanced Drug Delivery Reviews. 2021;179.
- Verbeke R, Lentacker I, De Smedt SC, Dewitte H. The dawn of mRNA vaccines: The COVID-19 case. Journal of Controlled Release. 2021;333:511–520.
- Ng’uni T, Chasara C, Ndhlovu ZM. Major Scientific Hurdles in HIV Vaccine Development: Historical Perspective and Future Directions. Frontiers in Immunology. 2020;11:590780.
- Duncan JD, Urbanowicz RA, Tarr AW, Ball JK. Hepatitis C virus vaccine: Challenges and prospects. Vaccines. 2020;8(1):90.
- Sriram S, Ranganathan R. Why human papilloma virus vaccination coverage is low among adolescents in the US? A study of barriers for vaccination uptake. J Family Med Prim Care. 2019;8(3):866.
- Durrheim DN. Measles eradication—retreating is not an option. The Lancet Infectious Diseases. 2020;20(6):e138–e141.
- Winter AK, Lambert B, Klein D, Klepac P, Papadopoulos T, Truelove S, et al. Feasibility of measles and rubella vaccination programmes for disease elimination: a modelling study. Lancet Glob Health. 2022;10(10):e1412–e1422.
- Seward J, Jumaan A. Chapter 40 VSV: Persistence in the population. In: Human Herpesviruses. 2007. Cambridge University Press.
- Pardi N, Hogan MJ, Weissman D. Recent advances in mRNA vaccine technology. Current Opinion in Immunology. 2020;65:14–20.
- Polatoğlu I, Oncu‐Oner T, Dalman I, Ozdogan S. COVID‐19 in early 2023: Structure, replication mechanism, variants of SARS‐CoV‐2, diagnostic tests, and vaccine & drug development studies. MedComm (Beijing). 2023;4(2).
- Teng M, Yao Y, Nair V, Luo J. Latest advances of virology research using crispr/cas9-based gene-editing technology and its application to vaccine development. Viruses. 2021;13(5).
- Dubé È, Ward JK, Verger P, Macdonald NE. Vaccine Hesitancy, Acceptance, and Anti-Vaccination: Trends and Future Prospects for Public Health. Annu Rev Public Health. 2021;42:175–191.
- Thomson A, Robinson K, Vallée-Tourangeau G. The 5As: A practical taxonomy for the determinants of vaccine uptake. Vaccine. 2016;34(8):1018–1024.
- World Health Organization. Ten threats to global health in 2019. https://www.who.int/vietnam/news/feature-stories/detail/ten-threats-to-global-health-in-2019. Accessed 19 April 2023.
- Lemoine C, Thakur A, Krajišnik D, Guyon R, Longet S, Razim A, et al. Technological approaches for improving vaccination compliance and coverage. Vaccines. 2020;8(2):1–29.
- Freeman D, Lambe S, Yu LM, Freeman J, Chadwick A, Vaccari C, et al. Injection fears and COVID-19 vaccine hesitancy. Psychol Med. 2021.
- Debnath N, Thakur M, Khushboo, Negi NP, Gautam V, Kumar Yadav A, et al. Insight of oral vaccines as an alternative approach to health and disease management: An innovative intuition and challenges. Biotechnology and Bioengineering. 2022;119(2):327–346.
- World Health Organization. Immunization Agenda 2030. 2020.
- World Health Organization. Hepatitis C. 2022. https://www.who.int/news-room/fact-sheets/detail/hepatitis-c. Accessed 19 April 2023.
of interest
are looking at
saved
next event